Integrated diagnostics data advance infill development
Integrated diagnostics is a well-known term in the medical profession. A doctor’s role is to combine blood pressure, MRI scans, and clinical observations into a proper assessment of the patient’s condition and subsequently advise on treatment. For a proper diagnosis, it is essential to analyze all available data in an integral way. Ideally, the oil and gas industry’s approach to data should not be any different.
Large-scale data accumulation is becoming more common in shale development. However, the industry struggles with a data dilemma. On the one hand, operators know that they have to gather data to learn and improve. On the other hand, the learning and analysis require so much manpower that often the collected data are left unexplored. If the cost of analysis cannot be decreased substantially, one might wonder, “why collect data in the first place?” Reveal Energy Services, a company that specializes in pressure-based diagnostics, has been pioneering workflows and software that can swiftly integrate many data sources. Reveal has developed a novel, data interactions and data visualization platform that enables engineering teams to collaborate and gain significant insight into complex subsurface dynamics.
An Alberta Montney operator and Reveal looked back at a specific set of accumulated data to learn more about these subsurface dynamics, to make substantial progress in interzone communications and the impact of faults on fracture-driven interactions (FDIs). An understanding of these responses is not limited to increasing production only, but also to cost-saving by reducing excessive proppant and water.
In this study, production data, perf erosion data, fault/seismic data, and drilling log data are all integrated with offset pressure analysis to diagnose FDIs accurately and react on future pads, accordingly. This workflow is demonstrated, based on the Montney development of 23 child wells and 17 existing producers from four nearby pads.
PARENT WELL OFFSET PRESSURE ANALYSIS
To begin this diagnostic integration, it is important to understand the pressure responses seen on the existing producers. As each stage is completed on the surrounding child wells, the parent well may feel pressure responses deviating from the pressure buildup normally seen on a shut-in. These responses stem from three key mechanisms of interaction: 1) direct fluid communication; 2) indirect fluid communication/migration; and 3) undrained rock compression.
Direct fluid communication (also called frac hits) is when there is a direct, highly permeable pathway established between the child well and the offset well. This causes a relatively large and rapidly developing pressure response. In the case of fluid migration, the fluid pressure front moves from the child well through a network of fractures and faults with relatively low permeability, making the pressure response observed on the offset well gradual and delayed with respect to the treatment time. An undrained rock compression response relies on a distinct lack of fluid mobility between the child and parent well, to leave a purely solid rock response from the shale itself.
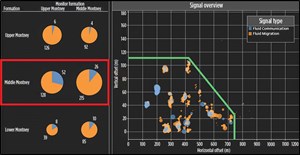
Offset pressure analysis provides a detailed look at mechanisms of communication between parent and child wells during stimulation. Aggregating these classifications over large numbers of stages builds the bigger picture as to what is going on in the subsurface. Figure 1 illustrates the frequency of response types from zone to zone, in addition to the distances at which these pressure responses are formed. A major learning from offset pressure analysis is well spacing constraints from the extent of the pressure responses seen.
Because of this, strategically placing wells in a specific wine-rack configuration and carefully scheduling the stimulation sequence may be most beneficial for future field development. The FDI counts from Middle Montney child wells to Middle Montney and Upper Montney parent wells are another learning from these results. Although in-zone communication between Middle Montney wells generated more responses, Middle Montney completions generated a higher probability of frac hits on Upper Montney parent wells, potentially resulting in a higher overall impact, in addition to a propensity in upward growth.
PRODUCTION DATA
Next on the list of integrated data is the use of post-frac production data on the parent wells, showing the initial and long-term impact from infill completions. Correlating pre- and post-frac production provides direct insight into the monetary impact from offset completions on each parent well. There are many factors that go into how a parent well will produce after nearby child well stimulations. Those include, but are not limited to, how many interactions there were over the course of the child well fracs, type of interaction, (i.e., direct hit or migration), completion technique of the parent well, formation of the parent well, and the age of the parent well.
To start, a simplified approach to production data is to classify the parent well as having one of three impact types from nearby stimulations: 1) constructive impact; 2) neutral impact; and 3) non-constructive impact. A constructive impact, while less common, can occur when hydrocarbon production increases after shut-in because of a pseudo-waterflood effect from nearby stimulations. More commonly, though, are neutral and non-constructive parent well impacts. It is also important to note that while there may be a neutral response on the parent well in production, the nearby child well may not be stimulating new rock, but instead lowering its respective SRV by simply restimulating the parent well. This situation, in turn, will still result in a net reduction over the course of the pad.
In this study, 67% of the parent wells had a neutral impact from nearby stimulations and all other showed non-constructive impact. For all non-constructively impacted wells, gas production had the biggest impact on total EUR. Condensate production, on the other hand, was only impacted on a single parent well. Water production was impacted on 56% of the parent wells and was always impacted when parent wells showed a significant number of offset pressure responses.
Figure 2 shows the daily and cumulative production from parent well P2 in the Upper Montney. This figure illustrates an initial drop in both gas and condensate production, in addition to a major jump in water production, post-frac. In about six months, condensate and gas production largely return to pre-frac levels. Going forward, an existing producer in similar circumstances could be protected best by focusing on mitigating direct fluid communication (as opposed to fluid migration). Measures taken on nearby child wells include the addition of diverter or more pinpoint stage placement with smaller stage spacing and treatment size, or both. This parent well had only been producing for approximately 300 days, leaving a large amount of potential recovery to be protected.
PERF EROSION DATA
Another diagnostic piece to the puzzle comes from perf erosion data. These data are obtained from a downhole ultrasound-based imaging tool that allows a view of the increased perf area generated from erosion during treatment. This area increase is correlated with a phasing direction in polar coordinates to determine which direction the slurry exited the wellbore and eroded individual perfs. The idea behind this information is that the more erosion seen on a perf, the larger the distribution of proppant-laden fluid into that perf. Cluster/perf efficiency on a frac job is never perfect, so having a tool that informs potentially where over-stimulated perfs are located can be beneficial to formulating specific solutions.
For this study, the phase direction that was directly in line with an offset existing producer was overrepresented in its perf erosion. What the perf erosion data have shown is that not only are existing producers acting as thief zones in the far-field environment, but that this imbalance is taking place in the near-wellbore environment also. The path of least resistance over the course of the entire stage leans in the direction of the parent well.
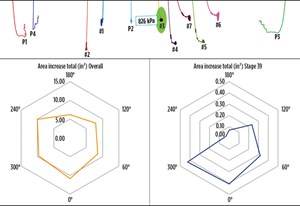
Figure 3 shows child well #3’s erosion-based, perf area increases on both the entire lateral and the individual stage, with the largest offset pressure response. The gun barrel view shows that child well #3 is directly offset to parent well P2 in the same Upper Montney layer, with P2 having a slightly higher average vertical depth. The data were presented with 0-degree phasing, starting at the bottom of the wellbore and rotating counterclockwise around the wellbore from a downhole perspective.
Based on the data, there is a clear preference for slurry to exit the wellbore between 240o and 360o in the southwest perspective, Fig. 3. This is in an almost direct path to parent well P2. Figure 3 also shows stage 39’s perf erosion data. This stage had the highest offset pressure response at 826 kPa. One would expect the largest pressure response on the parent well to correlate with high perf erosion in the direction of the parent well, and this seems to be the case. The 300° and 360° directions have clear phase bias on this particular stage.
In summary, for parent well P2, there has been 1) An impact on gas, condensate and water production from nearby stimulations; 2) A reduction in potential SRV from the nearby child well because of a highly fractured, pressure sink environment created by an existing producer. Now, with perf erosion data, additionally known is 3) An uneven distribution of proppant-laden fluid will also reduce the potential SRV to the other side of the child well (to the east based on Fig. 3). As more data accumulate, the subsurface picture starts to become more apparent.
SEISMIC AND DRILLING
The last diagnostic data sets integrated into the study were drilling and seismic data overlaid on interpreted faults from a bird’s eye perspective. Understanding the natural faulting structure is crucial in the Montney, as it is very prevalent and a driving factor behind migrating fluid. A web of relatively higher permeability highways across the reservoir can promote fluid flow over long distances to existing producers and their respective pressure sinks without ever creating net pressure or stimulating new rock. Also, a highly naturally fractured environment extends the distance of influence for treatment stages. While fracture half-lengths can reach upwards of 400 m, parent wells over 1 km away can repeatedly exhibit offset pressure responses. Faults, natural fractures, and existing fracture networks are the catalysts extending these areas of influence. Being cognizant of major faults and other secondary permeability sources can help operators be proactive at efficiently stimulating SRV at a stage level.
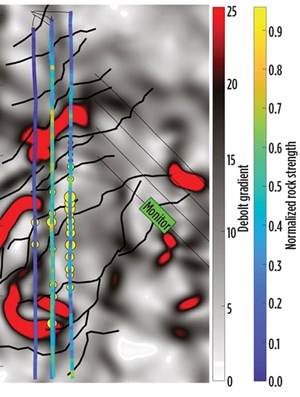
Figure 4 shows the plat view of a pad consisting of four child and four parent wells. The north-south wells on the left are the child wells, while the diagonal wells to the right are the parent wells. The background color represents the seismic Gradient in the Debolt, indicating the severity of the change in rock stress across the reservoir. A higher Debolt gradient value correlates with a higher likelihood of a fault present. The dark black lines overlaid are the operator’s internal interpretation of existing faults in the region, based on the seismic data available. The color gradient along the wellbore represents the normalized rock strength generated from the drilling logs. Lastly, the yellow bubbles on each child well stage are sized, based on the response magnitude seen on the parent well monitor.
When the child well stages are physically close to the parent wells, we see consistent offset pressure responses, but as stages are farther away, signals are more scattered. The common denominator of the scattered signals is an existing fault structure, allowing distant treatment stages a pathway to the parent well. Whether this pathway is directed to another existing child well fracture network, or the parent well fracture network directly, faults allow for long-range fluid mobility, which can result in parent well damage, in addition to a lack of stimulated rock surrounding the child well.
These data can be used to anticipate faults in the region and to react accordingly, whether that means using a diversion technique through mid-treatment diverter, high-viscosity sweeps, multiple proppant ramps (etc) or reducing stage slurry and proppant volumes to prevent inefficient proppant placement. If nothing is done and all stages are treated equally, then there is a potential for under-stimulation in stages, in proximity of severe faults.
FINAL THOUGHTS
To put all of this in perspective, the industry has always needed to integrate and analyze collected data. Historically, however, this has been a very labor-intensive, expensive process, in which files are loaded into, and transferred between, a multitude of different software programs. With this in mind, we can safely conclude that operators, for the most part, could not justify investing the required time in data analysis. The lost opportunity impact on development decisions is hard to quantify, but may be significant. Therefore, it is paramount that going forward, the industry unlock this data potential of lessons learned with the right workflows and tools.
There are, basically, two major schools of thought to break this data problem. The first is a machine learning or artificial intelligence approach, and the second is a more traditional engineering approach supported by dedicated software. Artificial intelligence and/or machine learning is faster and less expensive, but removing the human element in these approaches also removes the data quality guarantee that only human intervention can offer. This is referred to as a human-in-the-loop approach, and the key to success is to make the human intervention as efficient as possible.
To this aim, a novel data interactions and data visualization platform has been developed that enables engineering teams to collaborate and gain significant insight into complex subsurface dynamics. These renewed data interactions foster discussion and help to effectively communicate new views and ideas, and allow operators to uncover the knowledge capital stored in their data. The fusion of offset pressure analysis, production data, perf erosion data, fault/seismic data, and drilling log data enabled an integrated data study on FDIs in the Montney. Understanding how FDIs impact both the parent and child wells during infill well development is key to tackling the issue at hand.
This study has shown there is an impact in the near-wellbore environment manifested by a distinct pattern in perforation erosion toward an offsetting existing producer experiencing direct fluid communication. In addition, the impact on the far-field environment can be gauged through the impact on water/gas production from the parent well. Knowing this, the operator in the future can use preventive measures that mitigate these impacts and address the frequency of direct fluid communication FDIs. This vital information allows operators to continue infill development without restimulating parent well SRV or leaving untapped rock. Using integrated diagnostics helped to paint the bigger picture of what really was going on downhole, allowing future development to be efficient in both cost and production.
- Digital transformation/Late-life optimization: Harnessing data-driven strategies for late-life optimization (March 2024)
- The reserves replacement dilemma: Can intelligent digital technologies fill the supply gap? (March 2024)
- Digital tool kit enhances real-time decision-making to improve drilling efficiency and performance (February 2024)
- Digital transformation: Digital twins help to make the invisible, visible in Indonesia’s energy industry (January 2024)
- Digital transformation: A breakthrough year for digitalization in the offshore sector (January 2024)
- Quantum computing and subsurface prediction (January 2024)
- Applying ultra-deep LWD resistivity technology successfully in a SAGD operation (May 2019)
- Adoption of wireless intelligent completions advances (May 2019)
- Majors double down as takeaway crunch eases (April 2019)
- What’s new in well logging and formation evaluation (April 2019)
- Qualification of a 20,000-psi subsea BOP: A collaborative approach (February 2019)
- ConocoPhillips’ Greg Leveille sees rapid trajectory of technical advancement continuing (February 2019)