Using science-driven measurements to maximize soil-based carbon credits
Soil is widely acknowledged as a superior carbon absorbent, credited with helping capture more than three times the amount of atmospheric carbon dioxide (CO2) currently emitted.
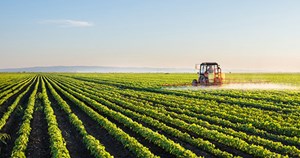
Accordingly, the carbon captured in agricultural activities within this natural CO2 sink provides landowners and farmers a potentially lucrative incremental revenue stream via the burgeoning carbon credit marketplace (Fig. 1), through which oil and gas companies leasing their acreage can buy credits to offset emissions from drilling and production operations. Investor-pressured operators, in turn, are afforded a practical and immediate mechanism to help advance the net zero push without the enormous investment and build-out lead times of more high-profile carbon capture, utilization and sequestration (CCUS) projects.
Conventional core sampling and diagnostic techniques, however, woefully under-quantify the volume of CO2 sequestered, leaving substantial carbon credits stranded and undocumented, thereby rendering them unavailable for sale to operators and other leasing entities. In the U.S, typical sampling entails one core/100 acres at a maximum depth of 1 ft, well above the root systems of most crops, which are natural carbon magnets through the photosynthesis biochemical process. This practice, thereby, fails to accurately account for deeper and high-resolution CO2 mapping, preventing either party from fully reaping the benefits.
Reflecting the effective transfer of oilfield technology to the agricultural nature-based solutions (Ag-NBS) space, a groundbreaking West Texas initiative, unveiled in conjunction with Permian basin asset development operations, has conclusively verified soil-based carbon sequestration levels 25 times higher/acre than those previously recorded.
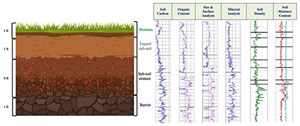
Along with more frequent and deeper sampling, the cores underwent a comprehensive testing protocol that capitalizes on advanced oilfield sub-atomic and nano-laser diagnostic expertise, Fig. 2. Abetted with specialized carbon mapping and particles diagnostic equipment, the suite of evaluations examines critical total carbon organic and inorganic content, as well as particle size and surface area distribution, crystallinity, geomechanics, density, porosity, moisture, and elemental analysis. As a result, the recently engineered CarbonTrace carbon verification and soil analysis technology, for the first time has definitively quantified the full breadth of carbon that can be captured and permanently contained in soil through associated agricultural activities.
NATURE'S CO2 VAULT
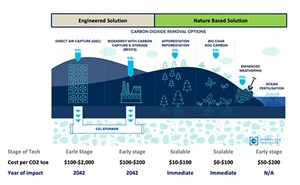
Since the signing of the Kyoto Protocol in December 1997, CCUS has rapidly evolved as a universally ubiquitous practice to help reduce greenhouse gas (GHG) emissions. More recently, a number of largely academic papers have dissected the capacity of agricultural land and forests to attract and permanently retain CO2, elevating nature-based capture and sequestration as a pragmatic alternative to often complex and high-cost CCUS projects, Fig. 3.
For comparison, the equivalent costs of sequestering CO2 through engineered removal options, such as direct air capture or bioenergy with CCUS, are estimated at anywhere from $100/ton to $2,000/ton of CO2 equivalent (toe) and can take nearly 20 years to impact atmospheric emissions. Nature-based solutions, on the other hand, are scalable from zero to a high of $200 per CO2/toe captured, with an immediate impact on companies' decarbonization goals.
In a withering assessment of the affect of engineered CCUS schemes on global net-zero emissions, the International Energy Agency (IEA) in October said a mere 20 commercial carbon-capture projects under development had managed to reach the Final Investment Decision (FID) stage.1 Even if all announced projects proceed, IEA projected they would provide roughly 40% of annual CO2 containment required to meet the global 2030 decarbonization goal of 1 gt/yr.
Soil, on the other hand, has been described as trailing only the ocean as the world's largest carbon storage vessel, estimated to contain 2,500 gigatons of CO2, or three times the volume in the earth's atmosphere.2 In the U.S., for example, an earlier assessment pegged agricultural acreage—comprising crop and grazing lands and forests—as having the capacity to sequester 650 million metric tons of CO2/yr,3 which could now be considered a conservative estimate. Importantly, 1 metric ton of carbon is equal to 3.67 metric tons of CO2.
Through the photosynthesis process, the root systems of crops and trees draw CO2 from the atmosphere, which is then stored in the soil carbon pool, commonly referred to as the soil organic carbon (SOC). Measuring and reporting current SOC volumes and the remaining absorption capacity for shallow and deeper soil can only be accomplished in a laboratory setting.
Along with SOC content, the non-exhaustive range of factors that affects the amount of CO2 that can absorbed by soil, includes soil type, the hydric content, rainfall, grade/slope, soil erosion, depth of root systems and specific land usage, with regenerative agriculture and managed grasslands generally said to achieve higher carbon storage capacity.
Consequently, accurate and high-quality measurement is required to ensure high carbon credit quality, which is an essential criterion for carbon trading and optimizing the value in the growing carbon credit marketplace.
NAVIGATING THE CARBON MARKET
Carbon credits, referred interchangeably as carbon offsets, have been characterized casually as “permission slips” that enable oil and gas companies, and others, to neutralize the Scope 1, 2 and 3 emissions resulting from their operations.4 Aside from California’s regulated cap-and-trade mandate, carbon trading in the U.S., for now, largely involves voluntary transactions, meaning operators can deal directly with landowners to purchase credits, providing the volume of CO2 sequestered has been measured and conclusively verified.
While prices/toe of sequestered CO2 fluctuate, the cumulative value of nature-based carbon capture solutions, specifically land use and forestry, has risen exponentially. Between 2016 and 2021, transaction values in the voluntary carbon marketplace are estimated to have increased 20 times, to a cumulative value of more than $1.32 billion.5 For clarification, one metric ton of CO2 validated as being sequestered translates into one carbon credit.
A number of entities have sprung up over the years to assist pertinent parties in navigating the loosely organized U.S. carbon credit marketplace. In Texas, the Bcarbon nonprofit, formed in 2019 by Rice University’s Baker Institute for Public Policy, has developed measurement-focused protocols, aimed at providing “a nature-based pathway to net-zero goals.” The Bcarbon Soil Carbon Protocol, for instance, defines methods for quantifying the increase in soil organic carbon achieved over time on a property “with the necessary statistical reliability to support the issuance and sale of carbon credits.”
To that end, the debut of the CarbonTrace technology in the West Texas pilot program was orchestrated to quantitatively validate the volume and duration of the CO2 sequestered beneath the tested acreage. The data-driven technology was deployed on multiple agricultural-related projects collocated with associated oil and gas activities.
To account for topographical and lithological deviations and heterogeneity, up to 25 core samples/100 acres were extracted, 24 more than the conventional sampling process. Unlike the traditional approach, samples also were taken 10 ft or deeper, well below the root systems of most crops and trees. As noted by Sothe, et al, evaluation at depth allows for a more adequate prediction of SOC concentration.6
The samples were subsequently delivered to QuantumPro’s Houston sub-atomic analytical lab, where each core was subjected to a seven-test suite of specialized data-driven diagnostics.
COMPREHENSIVE IN-HOUSE DIAGNOSTICS
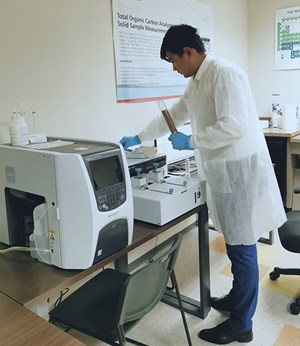
Analyzing soil carbon and organic content is arguably the most fundamental measurement of actual soil sequestration verification and quantification, as pointed out by the University of Aberdeen’s Pete Smith, et al, 7 and others. Dry soil combustion is regarded as the most accurate and versatile method of measuring the total carbon (TC), inorganic carbon (IC), and total soil organic carbon (TOC/SOC) of a soil sample, Fig. 4.
For this evaluation, the core samples are heated in an oxygen-rich environment to more than 680°C (1,256°F) inside combustion tubes filled with a platinum catalyst. The oxidation-generated CO2 is detected, using a high-sensitivity infrared gas analyzer (NDIR), with a detection limit of 4μg/L—the highest level for the carbon detection and quantification method for soil sample analysis. The determination of the inorganic carbon also can be carried out in a separate furnace in the module. The inorganic carbon in a soil sample is measured by pre-mixing the soil sample in phosphoric acid, with the resulting carbon dioxide purged at 200 °C (392°F) and measured using an infrared gas analyzer.
Soil moisture content analysis, likewise, is among the most vital measurements of CO2 sequestration capacity. A U.S. Forest Service-led academic investigation concluded moisture, rather than temperature or mineral content, is a key enabler to soils’ carbon sequestration capacity.8 As such, extreme drought conditions can seriously affect the capacity of soils to attract and retain carbon.
Simply put, moisture content represents the ratio of the mass of water held in the soil to the dry soil, which is determined by the difference before and after drying the soil sample. The moisture content influences the physical properties of the soil, including, but not limited to, weight, density, viscosity and conductivity. Whereas the dry mass of the soil particles is fixed, the amount of water within moist soil can vary. Therefore, to ensure consistency, moisture content is calculated on a dry basis, rather than a total mass basis.
This simple measurement, which is vital to determine topsoil moisture content to better understand soil characteristics, is typically followed with the soil proctor compaction test that measures volume and weight to determine topsoil compaction over time. Specifically, this widely used analysis determines the optimal moisture content at which a given soil type will become most dense and achieve its maximum dry density.
The corresponding soil density evaluation of a compacted soil sample is also an important test for soil characterization, as a particular density assessment is required for soil carbon sequestration analysis. Traditionally, a core sample is placed in a tube and weighed to determine how much soil, sand, or other fine material fits in each area with the current level of compaction. The materials can be weighed both dry and wet to provide additional information about their density.
Particle size distribution (PSD), which is a key consideration in evaluating bridging materials for oilfield drill-in and completion fluids, is equally important for assessing nature-based carbon sequestration capacity. For this analysis, soil samples undergo PSD and surface area analysis, using advanced nano-lasers to differentiate the carbon absorption capability of different soil lithologies and particle sizes. Determining the percentage of each size and surface area of the grain particles contained within a soil sample can subsequently be used to produce the grain size distribution and surface area curves. The resulting data are used to classify the soil, characterize the lithology and ultimately predict its behavior in a soil-based carbon sequestration sequence and total carbon sequestration capacity.
The nano laser diffraction analysis of the PSD and particle surface area of each soil sample measures the intensity of light scattered, as a laser beam passes through a dispersed particulate soil sample. Large particles scatter light at small angles relative to the laser beam, and small particles scatter light at large angles. The angular scattering intensity data are then analyzed to calculate the size and surface area of the soil particles that created the scattering pattern, using the Mie method of light scattering.
The all-inclusive diagnostics suite also entails soil compound and mineral analysis, which is performed, using advanced X-ray diffraction (XRD), a versatile, non-destructive analytical technique used to analyze physical properties, such as phase composition, crystal structure, and orientation of soil particles. This sub-atomic technique, which provides detailed data on the atomic structure of crystalline substances in each soil sample, is an extremely powerful tool for identifying compounds and minerals in rock and soil samples.
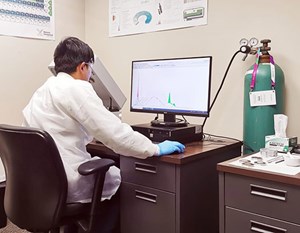
The related soil geochemical and elemental analysis uses sub-atomic energy-dispersive X-ray fluorescence (EDXRF) spectrometry to quantify the specific elemental composition and compounds in a core sample that affect the sequestration process and the ultimate sequestration capacity, Fig. 5. The non-destructive EDXRF goes beyond the average detection limits for many elements of soil geochemistry and elemental importance, which are well below 1 ppm.
PROJECT RECAP
For the West Texas pilot project, the advanced diagnostics conducted on the deeper core samples effectively quantified almost 10 times more certifiable total carbon by weight, compared to earlier shallower measurements. Complemented with the all-inclusive analytics, the increased sampling frequency and deeper depths translated to nearly 25 times more incremental carbon sequestered/acre and available for sale in the carbon credit marketplace, as compared to previous calculations when taking a single sample every 100 acres at 1-ft depths.
The procedure will be repeated yearly to account for higher-frequency sampling and the additional carbon sequestered during each new planting season. Insight derived from the project may also provide opportunities to modify soil and crop management practices to further enhance carbon capture and help oil and gas operators to drive the efforts to eventually offset all atmospheric emissions.
SELECT REFERENCES
- "IEA tones down hope for CCUS," Stuart Penson, Carbon Economist newsletter, Oct. 4, 2023.
- "Texas ranchers, farmers turn to carbon credits to keep fields, pocketbooks green," James Osborne, Houston Chronicle, April 13, 2003.
- "Farmers, Foresters Can Play Key Role in Managing Carbon," Soil Science Society of America, https://www.soils.org/news/media-releases/releases, April 9, 2009.
- "The Ultimate Guide to Understanding Carbon Credits," Carbon Credits.com
- "The Rising Demand for Nature-Based Carbon Credits," Carbon Streaming.com
- "Large Soil Carbon Storage in Terrestrial Ecosystems of Canada," Sothe, C., A. Gonsamo, J. Arabian, W. Kurz, S. A. Finkelstein and J. Snider. AGU Global Biodegradable Cycles, Feb. 2, 2022, https://doi.org/10.1029/2021GB007213
- "How to measure, report and verify soil carbon change to realize the potential of soil carbon sequestration for atmospheric greenhouse gas removal," Smith, P., J‐F. Soussana, D. Angers, L. Schipper, C. Chenu, D. Rasse, N. H. Batjes, F. van Egmond, S. McNeill, M. Kuhnert, C. Arias‐Navarro, J. E. Olesen, N. Chirinda, D. Fornara, E. Wollenberg, J. Álvaro‐Fuentes, A. Sanz‐Cobena and K. Klumpp, Global Change Biology, January 2020, 26 (1): 219-241.
- "Moisture-driven divergence in mineral-associated soil carbon persistence," Heckman, K., A. Possinger, B. Badgley and B. Strahm, Proceedings of the National Academy of Sciences, Jan. 3, 2023.
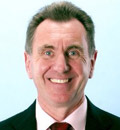
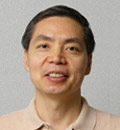
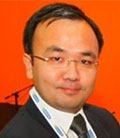
- Coiled tubing drilling’s role in the energy transition (March 2024)
- Advancing offshore decarbonization through electrification of FPSOs (March 2024)
- Executive viewpoint: TRRC opinion: Special interest groups are killing jobs to save their own (February 2024)
- Energy Addition is what’s required (December 2023)
- Buddy, can you spare a credit? (December 2023)
- Digital transformation: Accelerating productivity, sustainability in oil and gas (November 2023)