Exploration Report
Interest surges in seafloor node seismic systems
After years of languishing in scientific use, improvements in technology combined with changing oil industry needs have brought these systems to the forefront.
Perry A. Fischer, Editor
Four component cabled seafloor seismic systems have been around for about a decade. They will continue to provide cost-effective data acquisition, especially when multicomponent data is essential. However, increasingly, operators and contractors are asking, “Why not eliminate the cable?” But, with little or no field experience, judgment, logic, and just plain faith become the tools to choose the best seafloor system for a given application, as well as the right level of R&D investment.
Will operators see enough value in autonomous node systems to foster their development, even to the point of overlapping existing ocean bottom cable systems? Numerous tradeoffs in efficiency and cost immediately become evident. The raw technical tools have been around for several years, but ingenious designs and bold commitments by management will be needed to bring them to full commerciality. And what happens if, after much investment and development, operators just don't feel the value is there? Developing new seismic systems is not for the timid, but it sure is interesting.
BACKGROUND
It is surprising that no breakthroughs have been needed to achieve the latest crop of autonomous seafloor nodes, just the normal, incremental improvements in technology over time, good engineering and proof-of-concept, and of course, bold financial commitment. Batteries still supply the power, although they could be lithium ion rather than alkaline depending on costs and HSE considerations. Onboard recording devices are smaller and require less power, whether mechanical hard drives or solid state. Clocks – the essential ingredient synchronizing times among nodes for good imaging – are crystal driven and more accurate. And of course, good designs and materials for seals that must withstand 10,000-ft water depths are crucial. Creativity in accurate placement and retrieval make for interesting differences among systems.
Seafloor nodes have been used for more than 70 years. Generically known as seafloor seismographs, they have been primarily used for regional studies at basin scales, crustal investigations and deep-earth studies. For such uses, positioning requirements are relaxed, as is data density. Thus, sparse deployment is common. If artificial sources are used, high shot densities are generally not required. Their design often includes sensitivity to frequencies higher than those needed for oilfield applications, but there is considerable overlap.
In 1989, Eivend Berg embarked on a series of experiments to test the feasibility of acquiring shear wave data with seafloor sensors that were cabled back to a ship. Together with James Martin and Bjørnar Svenning, and supported by Statoil, they proved the viability of multicomponent seafloor acquisition for oilfield use in a shoot of the gas-occluded Tommeliten field in late 1993. Results were presented at EAEG (now EAGE) the following year. During the next few years, these experiments would result in the creation of a Norwegian company, Subseaco, which would further seafloor acquisition methods. Subseaco was eventually bought by CGG, and Berg and Svenning went on to form SeaBed Geophysical.
As deep and ultra-deepwater discoveries were increasingly becoming a reality, the challenges of cabled seafloor systems grew more problematic. The research direction focused on battery powered, autonomous seafloor sensors. The DEMO 2000 consortia comprised SeaBed Geophysical, SINTEF, Norske Hydro and TotalFinaElf.
Meanwhile, companies such GeoPro kept refining their seafloor seismographs, primarily for scientific use, but also for some oilfield clients.
In 2002, the IMPREDO Project (Statoil, Norsk Hydro, Total, NFR) was successfully carried out. The project was a 128-node multicomponent test over Norway's Volve field, acquired by SeaBed, which comprised sparse receivers (400-m spacing with 2-3 km offsets) for certain imaging problems and 4D repeatability. Also in 2002, BP contracted SeaBed to develop autonomous nodes capable of being deployed in 10,000-ft water. This contract may have been the final signal for other service providers to jump into the fray, or risk losing out on a developing market.
KEY DRIVERS
Autonomous nodes may be the only viable way at present to acquire multicomponent data in certain environments. This does not mean that conventional cabled-seafloor acquisition is impossible in these areas. In fact, some companies haven't given up on ocean bottom cabled systems, not even for ultra deepwater. OBC still offers a variable sampling ability, even high density, and long offsets, and pressure ratings are not a problem either. But more ingenious deployment and retrieval methods, and dealing with the problematic connection to surface, are needed in order to make these systems feasible below 1,500 ft. Spools, baskets, tractors and other devices for deployment/ retrieval are on the drawing boards, and a subsurface recording station may provide a hybrid system, but none of these are actively being built to the author's knowledge.
For now, it appears that, in an industry where contractors and manufacturers are grateful for any R&D help they can get from oil companies, the majors – certainly BP – have chosen to advance autonomous nodes for many of the reasons discussed here.
For 4D applications, the number of repeat surveys determines cost effectiveness when compared to permanently installed cabled systems. After some number of repeat surveys, the cost of a permanent seafloor cable system is absorbed, since re-acquiring data only calls for a shooting vessel, which may be a permanent standby field vessel. With nodes, perhaps less than 10 surveys will still be more cost effective than a permanently installed OBC system. Another aspect of nodes versus permanent OBC is the survivability of the cable system. In both respects – the value of many repeat surveys and survivability of equipment – all eyes are on the Valhall LoFS permanent system. Fortunately for the industry, BP has partners Amerada Hess, Shell and Total, all of which can benefit from whatever lessons are learned there.
Node systems are ideal when there is a need for better imaging (illuminating) under overhangs and geobodies (e.g., salt structures), where wide apertures matters most. In addition, the multicomponent aspects of both OBC and seafloor node systems could be essential in the case of gas-occluded reservoirs, but nodes might offer improved economics.
As mentioned above, certain areas lend themselves to the solutions offered by seafloor nodes. These places are areas of seafloor obstacles, whether from development infrastructure (e.g., flowlines, pipelines, moorings) or from a naturally rugose seafloor (e.g., furrows, ledges, pockmarks). More important are deep and ultradeep waters, say, more than 1,500 ft, certainly more than 6,000 ft, where multicomponent seismic acquisition has not kept up with drilling technology.
Better vector fidelity might be claimed relative to cable systems, since there is no interference from the cable. However, this may now be more of a theoretical problem than a real one, with new cable designs that decouple the sensor from the cable.
Improved repeatability for 4D can be claimed for nodes, but only in the case of highly accurate positioning methods. However, it should be noted that if OBC were also positioned by the same expensive, labor-intensive methods (e.g., crane, ROV, LBL ranging), repeatability would be similar. And there are not yet 4D surveys that prove the claim.
Sparse – receiver cost benefits. The cost benefits of node systems are a key driver. Sparse sensors combined with reasonably high shooting density yields a relatively large, multi-azimuth wide-aperture data set that covers a large area of seafloor efficiently. Costs are lower when the survey occurs as a single patch or, in any event, fewer patches than with OBC, and when the survey is much larger than a conventional-sized (5 km X 1 km) OBC patch. This results in fewer shots per unit area surveyed and fewer node relocations. However, although the survey duration will increase with the number of patches, owing to the time it takes to place and retrieve each node for each patch, the number of sensors per unit area is likely less relative to OBC. This is not necessarily a bad thing. The amount of data mapped into each receiver, or fold, can still be reasonably high, just by shooting at a normal, every 5-sec. density. Also, high-density sensor networks may not be as important for subsalt work, where wide aperture is the overriding goal.
As the distance between nodes increases, each node becomes increasingly important. If a node is located in a crucial spot for subsalt illumination, loss of that node, either through equipment failure or inability to retrieve, can put a big hole in your data. High-dollar placement and retrieval systems should eliminate mis-location. Reliability will have to be proven.
Logistical considerations must be kept in mind. The survey must be completed within the expected lifetime of the batteries. For very large areas that are to be shot as a single patch, a separate, additional power pack can probably be supplied, but at an additional cost and with logistical complications. Although the cost benefits of single-patch acquisition increase for any size survey, these benefits could decrease for surveys requiring very large numbers of nodes, when certain logistical thresholds are reached, such as adding another vessel, needing increased deck space for node storage, adding crew members and so on. When such thresholds are reached, they must be carefully weighed against a pickup-and-redeploy scenario.
An analysis by Jerry Beaudoin and Graham Openshaw shows that the economics will always favor single-patch acquisition over any other mode, Fig. 1. This includes the investment cost of increased node inventory. However, practical considerations such as the logistical constraints mentioned above must be factored in. This allows survey duration relative to the number of nodes to be calculated, Fig. 2.
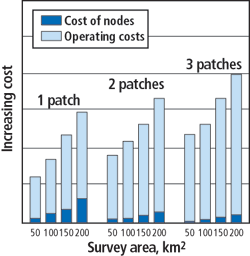 |
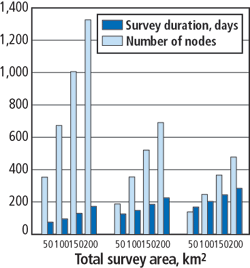 |
Fig. 1. Seafloor nodes: operating costs swamp equipment costs, while single-patch acquisition is always cheaper for a given area. From G. Beaudoin and G. Openshaw, “Gathering wide-azimuth ocean bottom seismic in deepwater: cables or nodes?” EAGE 66th Conference and Exhibition, Paris, June 7-10, 2004.
|
|
Fig. 2. Number of nodes and patches and their effect on survey duration. From G. Beaudoin and G. Openshaw, Ibid.
|
|
In addition, investment in node capacity has a different meaning for contractors than for oil companies, for they are the ones that must decide on how much CAPEX investment to risk. The trade-off here is that stockpiling thousands of nodes will result in lower-cost surveys and, therefore, more competitive bids. But thousands of nodes, if left at the shop, still cost money, and these costs necessarily must be passed on to operators. It is a difficult decision, based on predicting just how popular this acquisition mode will become.
The industry has just been through a similar CAPEX decision, where the cost benefits of ever-larger acquisition footprints drove vessel designs to higher streamer capacities in numbers, lengths and spreads, some up to 16, even 20 streamers, up to 6,000-m long covering acquisition footprints up to 11 km2. But that capacity often goes unused. As we have seen, along comes an ultra-low cost, four-streamer modified fishing vessel with new equipment, and beats everybody's bid.
DEPLOYMENT AND RETRIEVAL
With the least expensive, toss-it-over-the-side system available, such as the one from GeoPro, loss can and does occur. In shallow water, the positioning error is not too great, but in deep water, the error could grow to hundreds of feet. Still, that may be acceptable for certain basin and crustal studies. A weight carries the node to the seafloor. At retrieval, an acoustic signal releases a weight, allowing surfacing through buoyancy. Surface location is through radio and visual sighting of a flag and light flash. The company reports that the overall number of nodes lost by this method is about 1% (see Table). It is quick to add that these losses can be reduced to zero through the use of an ROV, assuming the client is willing to pay for it.
|
Surface retrievable (buoyant)
nodes. |
|
|
|
Number
deployed |
Number
lost |
|
|
|
|
|
|
93 |
0 |
|
|
|
160 |
2 |
|
|
|
73 |
0 |
|
|
|
66 |
1 |
|
|
|
300 |
4 |
|
|
|
30 |
0 |
|
|
|
40 |
0 |
|
|
Totals |
669 |
7 |
|
|
Emergency retrieval packages may be added to any node, in a manner similar to what is available with conventional seismic systems. On signal, these cause a tank to inflate a buoy, carrying the node to surface.
ROV deployment and retrieval provide the ultimate control, but at the highest cost. As water depth increases, the time it takes an ROV to resurface becomes too great, so stay-down systems are being developed. These center around baskets, sleds and tractors, all of which carry many nodes along the seafloor, deploying them at specific sites with an ROV.
POSITIONING
Several positioning options exist, with costs increasing with the desired accuracy. If there is any chance that the survey will become a baseline for future 4D work, accuracy will probably need to be sub-meter. Local seafloor effects can also tighten positioning requirements. These would include heterogeneous environments such as a mixture of hardgrounds and soft pockets, furrows, ridges, vents and other variations where even a few feet of positioning difference could resulting in false differences in the repeat survey.
Even for homogenous settings, tight positioning is highly desirable. Experiments with ocean bottom cables have shown that simply raising and redeploying the OBC a few hours apart, even in shallow water, can result in a false difference cube.
Companies such as Sonardyne specialize in underwater positioning. Nodes can be GIS referenced. The basic idea is to triangulate from three or more sources on the sea surface, each GIS located, to the seafloor. In ultradeep water, error from temperature differences in the water layers must be compensated.
Various baselines technologies, both long (LBL) and short (SBL, USBL), enable triangulation, first from the sea surface via GIS referenced points on ships and possibly buoys, then to three or more seafloor- mounted acoustic sources. From these, highly accurate positions can be worked out. Either the deployment vehicle, ROV or the nodes themselves can be accurately positioned. In addition, the nodes can have attached acoustic transponders that allow a dense acoustic network to be established as more nodes are deployed, which can greatly reduce error. The most accurate positioning for 4D would require leaving a marker on the seafloor that can be relocated acoustically much later.
THE CONTRACTORS
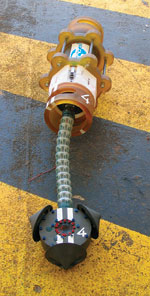 |
Fig. 3. Sercel's ARMSS 4C node.
|
|
Sercel's ARMSS (Autonomous Reservoir Monitoring Seismic System) 4C node is a fully autonomous seismic node that can record 4C data over an extended period of time. The Sensor Head (Fig. 3) has been designed with coupling to the seabed in mind, resulting in seismic data with high vector fidelity. A data logger records seismic and ancillary data to a local hard disk. The disk capacity allows recording periods of up to 45 days of 4C, 2-ms sampled data.
The data logger sampling clock is derived from a Digital Temperature Controlled Crystal Oscillator (DTCXO). The DTCXO compensates for clock frequency variations due to temperature variation (the prime cause of non linear clock error). Removal of linear clock variation on node recovery allows for accurate absolute sample recovery. The node is modular, allowing the use of different battery technologies and capacities. Both rechargeable and non-rechargeable battery technologies are supported. This node system was successfully tested in the North Sea and in the Gulf of Mexico. It is currently on a field test offshore West Africa.
Fairfield's Deep Z-Pod is a 4C autonomous node with dimensions of 22.5 in. by 10-in. high, Fig. 4. Weighing 200 lbs in air, it has a 28-day battery life and stores data in a flash memory. It is placed on the seafloor by a sled and ROV combination, designed and made by Sonsub. The two companies recently completed successful offshore sea trials of the Sonsub Deployment system and the Deep Z-Pod nodes in the Gulf of Mexico. The Deployment Skid System is described as an under-slung ROV-mounted skid, designed to mount directly under the company's 200 Hp Innovator ROV. Once deployed, the ROV transports the skid carousel system and the nodes to the seafloor. Both the skid and nodes are depth rated to 3,000 m (although the company also has a shallow-water node version). The skid is also used to recover the nodes when the survey is completed.
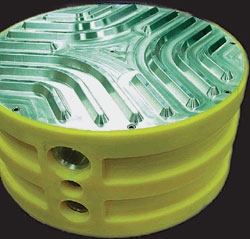 |
Fig. 4. Fairfield's skid deployed Z-Pod, It's a heavyweight, at 200 lbs.
|
|
K.U.M. is a German-based (Kiel) company that offers three different node systems. Although primarily for regional, basin scale and scientific, their frequency responses (4.5 to 100 Hz) and designs also allow for oilfield work. While they could be placed by ROV, the node is designed to be dropped overboard. An anchor weight, which remains on the seafloor, carries it down. Retrieval is by an acoustic signal from the ship, causing the weight to be released and the node to surface, where it is located by radio, flash and flag. Shown in Fig. 5 is the company's SLA-OBS model, a 4C autonomous node. The unit is rated to 6,000 m depths and can sample and record data continuously at a rate of 2 ms over 14 days.
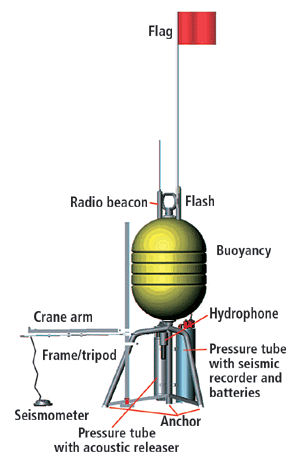 |
Fig. 5. K.U.M.'s weight drop deployed node seeks to prevent any noise or anisotropy by placing the 3C geophone up to 3 m distant using a crane arm.
|
|
GeoPro is a Hamburg, Germany-based company that offers seismic services to both institutions and oil companies. It specializes in nodes and wide-aperture imaging, having done so for the past nine years. The company's 4C SEDIS nodes are deployed over-the-side and retrieved similar to the K.U.M. units. The frequency response is identical to conventional 3C geophones in the 4.5 to 90 Hz range. The unit is rated to water depths of 6,700 m. D-size batteries provide power, as shown in Fig. 6. The latest SEDIS version has a 24-bit, 6-channel recorder, 20 – 30 GB hard disk that can record continuously up to 30 days, sampling at 2 to 16 ms. The instrument weighs 93 lb in air, including batteries. Another option with this unit is the use of six-channel, vertically suspended-hydrophone arrays, laid out either along a profile or in a 3-D grid.
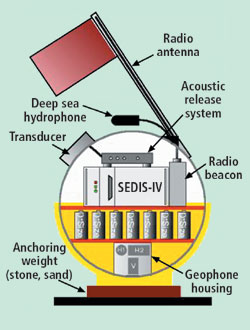 |
Fig. 6. GeoPro's autonomous node, although frequently updated, has been used in the oilfield for many years.
|
|
SeaBed was established in 1997. As is evident from the discussion above, in Background,the company's history is intertwined with the development of seafloor nodes in the past 15 years.
From 1997 to 2000, the company was involved with the ROKVA project at its test site in Norway, which was supported by BP, Norsk Hydro, ENI-Agip and the Norwegian Research Council. ROKVA was an extensive, comparative test of ocean bottom systems of cable, plate and nodes. During the past year, together with BP, the company conducted its first test of nodes in the Gulf of Mexico, followed by another test over the deepwater Atlantis field.
Further south, this year, PEMEX contracted the company to shoot Cantarell field in a survey that lasted four months, with 400-m spacing and 3-4 km offsets, using an ROV vessel for placement in 60-m waters. It is the largest ocean bottom 4C shoot in the world, comprising 1,400 node positions, Figs. 7, 8. Avoidance of infrastructure was a concern over the 230-sq-km receiver spread. The survey used a combination of a crane and ROV for precise placement.
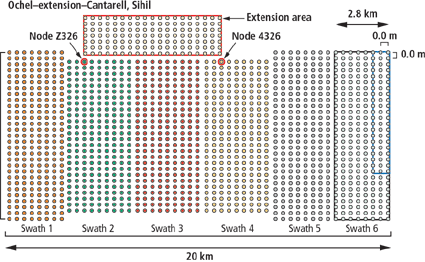 |
Fig. 7. SeaBed's Cantarell Project had about 230-sq-km receiver area with long shooting offsets. Narrow box in upper right corner of Swath 6 illustrates node economics. It is equivalent area for OBC acquisition (4 – 8 cables, 6 km each).
|
|
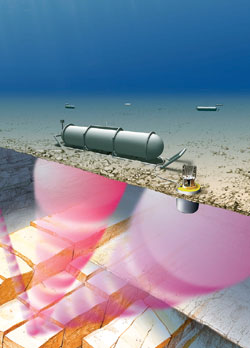 |
Fig. 8. SeaBed's design for autonomous nodes used in Cantarell Project.
|
|
The company has a new CASE design for 3,000-m water depths, and has just tested 6 prototypes in ultradeep waters in the GOM. The data was being processed at press time.
CONCLUSION
No one can say how this technology will look five years from now, which is what makes it all the more exciting. BP has reportedly made a tender for multicomponent seafloor node acquisition for one of its deepwater Gulf of Mexico projects. But it will take more than one major oil company to justify the investment now ongoing in node systems. Using multicomponent for reconnaissance seems to be a nearly dead issue. But that may not be true of node systems, if costs can be made substantially less than OBC. That remains to be seen. For now, this latest technology upgrade is aimed at oil and gas prospects and new fields, to delineate, appraise and add reservoir understanding and, with appropriate positioning care, as a baseline for future 4D surveys. It's just possible that, someday, this will be the way that all deepwater fields are characterized. 
ACKNOWLEDGMENT
The author thanks Steve Mitchell and Paul Bourgeois of Fairfield; Michael Briggs of Sercel; Rodney Calvert with Shell; Jerry Beaudoin with BP; Dimitri Ilinski with GeoPro; and Larry Denver, consultant for SeaBed Geophysical, for enlightening discussions on the topic.
|